Psicologia Generale Anole Pdf Merge
IntroductionThe perceptual world is the product of complex building strategies able to organize fragments into structures or to reorganize a structure into a different one. Sometimes these processes originate from a small variation in figural or luminance cues.A curious example is depicted in Figure where a white to black gradient background makes the silhouette of a Greek statue to appear.
In this figure the same set of irregular shapes is reproduced twice: on the left against a white background, on the right against a faded mid-gray. This change generates strong cohesive “forces” since the meaningless group of shapes depicted on the left transforms into a complex figural organization on the right. The silhouette of the “Winged Nike Victory of Samothrace” (standing on the grand staircase landing on the Louvre museum) is perceived against a dark gray background partially obscured by a shadow cone.
(A) Left side: irregular shapes against a lighter background. Right side: a replica of the pattern of irregular shapes drawn on the left side against a background of a mid-gray shade.
Note that the shapes are lighter or darker than the background. The spatial and photometric relationships follow the building schema of the “argyle illusion” so as to elicit the impression of a percept organized into two layers: an opaque figure, i.e., the Greek statue known as the “Winged Victory,” and a translucent filter, i.e., a shadow cone obscuring part of the statue's silhouette. The two contours and their contrast polarities (CP) are symbolized in the outlines in (D). Note that in the space embedded within the two profiles (invariant CP axes) positive induction signs converge in the cone and negative induction signs converge in the statue. (B) Enlargement of a portion of the leftmost pattern and the outline representation of the corners nearby with the “+” and “−” symbols indicating the lighter and darker sides of the edges (CP).
A double-reversing X-junction appears, so-called because the prolongation of a corner side meets an edge with the opposite CP. The CP persists, but along the corner perimeters.
No edge merging is expected in this condition. The contours we perceive coincide with the perimeters of the irregular gray shapes. (C) Enlargement of the correspondent portion in (B) following the darkening of the background.
A double-preserving X-junction is depicted, so-called because the prolongation of the corner edge encounters an edge of the same CP. We assume that in this condition the edges of two opposite corners perceptually merge as we have shown in the crossing schematics. The outline drawing symbolizes the result: the two acute angles of (B) are now reassembled as two intersecting linear edges.
In other words, the transformation of a double-reversing to a double-preserving X-junction involves a figural reorganization such that two adjacent corners are perceived as two intersecting edges. The layered representation of the image is not predicted by rules that apply in a context of single-reversing X-junctions. (D) The two contours of invariant CP resulting from the cohesive events described in (C). Note that the two contours have an opposite CP. Within the shadowed surface the symbol “−” indicates a propagation of darkness signals (the shadow appears darker than the background). The inside border of the “winged victory” is traversed by “+” signals (the statue looks lighter than the background). When superimposed these contours will generate induction signals of the opposite sign within the same area.
This organization is rather complex and results from contours and surfaces completion. Furthermore, it appears stratified in different layers, one for an opaque white figure and the other for a transparent dark filter (shadow). In the region where they overlap a luminance scission occur: the gray surface seems to illusorily split into a bright and a dark layer.
It is a further demonstration that the brightness (1) illusions, transparency and tridimensional organization of the percept are interrelated percepts.The “optical effects” of configurations such as the “winged victory,” i.e., light and dark shapes (inducers) alternating in shade and position at the opposite sides of an imaginary line, were first illustrated by the Bauhaus designer Joseph Albers. We have discovered the importance of these illusions in vision science thanks to the studies of Adelson who created a series of astonishing demonstrations.
The “argyle” and “snake” illusions are two of the most vivid demonstrations of brightness alteration arising in the perceptual world. Observers asked to describe patterns of alternating light/dark and dark/light columns of inducers (“argyle illusion”) reported the impression that portions of the background appeared darker and covered by a light filter whereas other portions seemed lighter covered by dark filter. These reports indicate a decomposition of the image into two layers: one for a reflectance and the other for a luminance (translucent) pattern. Adelson argued that the altered brightness of the background was the outcome of the “process of discounting the overlying filter” to recover background surface reflectance.Other researchers based their work on the key assumption that brightness alterations may originate in an image stratified into layers. Two main goals were pursued: (i) To unveil the computations at the origin of the decomposition into layers; (ii) To show the ways the scission can induce transformations in brightness.The computations underlying this decomposition depend critically on the boundaries between the targets and their surrounds; according to the visual cues used to identify the presence of non-uniform are patterns of four convergent surfaces or X-junctions. The cross point may coincide with a contrast polarity reversal in both the intersecting contours (double-reversing junction). In this case no transparency percept arises.
The contrast polarity may reverse in one direction only (single-reverse junction) or persist in both directions. These patterns are consistent with a transparency interpretation: unidirectional (unique) in the former case, bidirectional in the latter.Anderson and colleagues (; ) demonstrated that the scission into layers requires a more complex computation, an algorithm by which to process the geometric continuity, the contrast magnitudes, and their sign. Presented an algorithm detecting X-junctions, their contrast polarity, the circuits of polarity preserving X junctions, and ending with a decomposition of the image into layers.In a stratified image both the opaque and translucent layer may show an alteration in brightness (,;;; ).
The induction effects are opposite in the two layers: if the transparent layer dims, it appears to overlie a brighter opaque image; if the upper layer lightens, a dimmer surface is seen beneath.The claims that the brightness illusions such as the “snake” and “argyle” occur through interposition of a transparent layer have been confuted by several authors who demonstrated the persistence of the illusory phenomena despite the removal of the conditions for transparency perception (;;; ). Furthermore, a presence of a translucent layer may not entail a brightness alteration. Proposed an algorithm for computing lightness that does not assign a direct role to layering in brightness alteration. Further criticism of the layered representation approach has been reviewed. More recently, the image decomposition mechanism has been challenged by.All these findings suggest an approach to Figure illusions different from the “image decomposition and discounting mechanism.” There is no doubt that the right image appears as stratified in a translucent and a reflectance pattern but it is not a foregone conclusion that the layering is the cause of the brightness alterations. Nothing prevents us from thinking that the brightness illusions and the split into layers are contemporary events arising from the effects of a common basic phenomenon.
Such a conjecture can be tested only through a deeper reconstruction of what occurs when edges are integrated to form a contour. When, as in Figure, the sides of the irregular shapes reassemble to appear as the “winged victory” the phenomena of brightness alteration are likely to be present and contribute to the layering effect. The main purpose of the present work is to document the interaction between the phenomena of contour integration and brightness induction. The properties of these integrated figural units have been explored only in part and new data will contribute to the understanding of the vivid illusory effects that have been documented by Adelson and successive researches. A Contour Preserving the Contrast PolarityThe straight illuminant border in Figure (the shadow cone), like the filter borders in similar patterns, has been considered a source of essential information for the computations to generate a layered image. The crucial role should be played by the X-junctions, a pattern of four converging surfaces that convey the information necessary for the split into layers (see Figure caption).But a simple configuration confirms the criticism of those who questioned this role. In Figure a layered representation appears in violation of the geometrical constraint that has to be obeyed in order to evoke a phenomenal transparency.
Small rectangles of a different size and gray shade are arranged in irregular rows. Each dark rectangle is spatially contiguous to a lighter one but separated at the corners by a gap of variable length. In Figure the same configuration of light and dark gray rectangles is depicted twice: against a white background (Figure ) or against a mid-gray surface, or a surface of intermediate luminance between the shade of the dark and light rectangles (Figure ). (A) Rectangles of different sizes arranged in irregular rows against a background of a lighter shade. (B) Enlargement of a portion of (A): “+” and “−” indicates the lighter and darker sides, respectively.
Note that the central edge inverts the contrast polarity (CP) with respect to the flanking edges. (C) The reproduction of (A) with the background luminance intermediate between the dark and light rectangles. The rectangle corners are not in contact so that no X or T-junction results. Despite the spatial gaps, an irregular contour seems to interpose between the light and the dark rectangles to form a border appearing like a dark veil overlying a surface divided into light and dark regions. (D) Enlargement of a portion of (C) illustrating the edges CP and the genesis of a contour (or axis of invariant CP).
The edges are of the same CP so that they are expected to join. The doted curved lines symbolize the path of edge conjunctions. These local cohesive events sum to give rise to an overall effect that will be perceived as the border of a translucent surface. (E) Enlargement of a portion of (C) illustrating the edges and the genesis of a second contour of invariant CP. The doted curved lines symbolize the path of edge conjunctions. These local cohesive events sum to give rise to an overall effect that will be perceived as the border of the opaque surfaces. The variation in background luminance produces the same effects observed in Figure: the separated figural units organize into a stratified image in which opaque and translucent layers are visible.
Dark and light bands horizontally traverse the whole configuration as transparent filters; their borders follow the irregular pathway separating the dark rectangles from the light ones.Also the background appears to be divided into zones of different grayness, but, differently from the horizontal bands, which appear as opaque surfaces. When covered by a light veil they look darker; when covered by a dim veil they look brighter (see Figure caption).Therefore, in Figure we see a replica of the “argyle illusion” effects, i.e., brightness alterations of a layered image. Nevertheless, a question arises in regard to the relationships between the layering effect and the brightness alteration. The former has not a clear origin in Figure because the necessary cues to decompose the image into layers are not drawn: both X and T-junctions are absent as well as occlusion cues. The genesis of contour of invariant contrast polarity in Figure. (A) A portion of Figure vertically reoriented. (B) The two binding phenomena in the corner regions of Figure.
The dotted lines indicate the conjunction pathways. (C) The dotted line indicates one of the two axes ( axis 1 hereafter) of invariant CP resulting from the chaining of the local binding effects in the corner regions. (D) A dotted line follows the outer perimeter of the rectangles to indicate the second Axis of invariant CP ( axis 2 henceforth) resulting from the chaining of the local binding effects: the horizontally directed ones in (B). (E) The rectangles in (A) are occluded, at their extremes, by irregular shapes of opposite contrast polarity.
The reversal of the contrast sign inhibits the chaining process so that only the axis 1 survives. (F) The overall result of binding effects after the manipulation illustrated in (D): the vertical axis 1 and horizontal binding effects that do not integrate to complete an illusory contour. The hypothesis is that both the axes are sources of brightness induction effects. The appearance of this unit in the perceptual field is accompanied by a phenomenon of brightness/darkness induction: we perceive a dimmer gray shade on its negative side and a brighter gray shade on the opposite side.In other words, we suppose these contours of invariant CP to behave as a source of brightness induction signals as the continuous edges prove to be when the so-called process of edge integration occurs (;; ).Testing this hypothesis requires two steps.First of all the conditions must be reproduced that allow us to observe the brightness induction of one axis acting in isolation. Second, we have to document what happens when two axes of invariant CP give origin to opposite polarity signals in the same region of perceptual space.This overlapping of signals of opposite CP is illustrated in Figure. In Figure the axes of invariant CP delimit quadrants in two of which “+” and “−” signals diffuse and overlap. Let's consider the left half of the background in Figure.
It is passed through by negative signals induced by axis1 (Figure ) and by positive signals from axis 2 (Figure ).A simple stratagem allows us to disentangle the induction effects of axis 1 from the ones propagating from axis 2. This is illustrated in Figure. Irregular shapes are superimposed on the outer extremes of the rectangles so that the inducers are split into two halves of approximatively the same area and opposite gray shade with respect to the intermediate gray surround (“twin-shade inducers” from now on). This variation leaves the CP along the axis 1 unaltered, whereas the pathway along the external perimeter of the shapes reverses the CP several times.
Consequently the axis 2 cannot complete as Figure illustrates.The consequences on the brightness perception are not vivid in Figure but if the same configuration is inserted in a repetitive pattern they clearly emerge.In Figure different configurations of inducers have been created so that to allow the comparison of the effects generated by or two interlaced contours of invariant CP. Inducers with a jagged contours were drawn in order to put a further obstacle to the rise of the phenomenal transparency. The three configurations have been depicted against the same mid-gray homogeneous background, therefore whenever light and dark region are perceived a brightness illusion is generated.
The three figures have the same homogeneous gray background. (A) Columns of jagged squares alternating in shade at the opposite side of a vertical dividing line. The building schema is the same as the Adelson's “argyle illusion.” Note the illusory alternation of dark and light gray regions. Opaque regions similar to piles of road signs alternate in dark and light gray shades. Vertical bands alternate as shadow or illuminated zones.
(B) Columns of irregular shapes split into dark/light surfaces are used to generate vertical invariant CP axes. The basic drawing is identical to (A), irregular shapes have been superimposed on the squares so that the CP reverses along their outer contour.
Psicologia Generale Anole Pdf Merger
The vertical axes are indicated by the bidirectional arrows. The perception of alternating dark and bright bands on the background is illusory, since they have the same luminance. Note that the background appears to dim when embedded between +/− −/+ axes and to brighten when embedded between and −/+ +/− axes. Labels D and L indicate the regions between +/− −/+ and −/+ +/− axes, respectively, and are regions that appeared as dimmed or lightened, respectively, in the test condition “divided background” (see text). (C) The same as (A) but with inducers on the left of the column mirror-imaged.
Whatever pathway is followed, the CP inverts periodically. The arrows below point to the axes of variable CP (±). Labels D and L indicate the corresponding regions that were progressively darkened or lightened when the figure served as comparison stimulus. In Figure the inducers are aligned along a straight line alternating in gray shade and fall on opposite sides of an imaginary line. As in Figure the CP across this line is invariant, consequently the aligned sides are predicted to bind. A second contour of invariant CP is assumed to conjoin the inducers outer perimeters ( axis 2). Each column of inducers alternate with a column of opposite CP (see Figure caption).The same configuration is reproduced in Figure with a crucial variation in the inducers surface: they are split into two halves of approximately the same area and opposite gray shade with respect to the intermediate gray surround.
This variation left the CP along the vertical a xis 1 unaltered, whereas the pathway along the external perimeter of the shapes periodically reverses the CP. Therefore, only one source of induction is active in Figure, the vertical axis 1.In Figure even this source is inhibited since the inducers are reoriented so that to reverse the CP along both the two directions of contour completion.If we compare the gray backgrounds, a striking first datum emerges: the background that in Figures appears divided into dark and light regions is seen without differences in brightness in Figure. This supports the hypothesis that the presence of axes of invariant CP coincides with the brightness alterations.The role of the axis 1 of invariant CP is documented by the Figure. Here the twin-shade inducers have inhibited the axis 2 completion while leaving the axis 1 unaltered. Note the alternation of dark and light vertical bands in the background. We can check that the direction of the change in polarity is predicted by the CP of the axes.
Their locations are indicated by the bidirectional arrows and the CP polarity by the “+” and “−” at the opposite sides. For example, a background's illusory brighter region is embedded between −/+ and +/− pairs of axes.The same axes ( axes 1) complete in the top figure (the bidirectional arrow symbolize this correspondence) but in concomitance with the axes 2.
The conditions now exist for the observer to make direct comparisons of the effects generated by two axes or by one only.If we concentrate on the leftmost columns we can check that it appears to brighten in Figure whereas a dark gray seems to fill it in Figure. Nevertheless, the reversal of polarity induction is to be associated with a second evident illusion in Figure: alternating vertical bands of different illumination. Between the leftmost two columns a rectangular beam of light is projected on a surface in which a surface bordered by a square waved contour is colored of dark gray.This can be considered the “layered image” solution when a region of the background is passed through by induction signals of opposite CP.
The solution emerges despite the violation of the geometrical constraints for the transparency to arise and confirms the role of the perceived transparency, or layering, in modulating brightness. The illusory alternation of light and dark regions separated by square-waved contours is striking, more vivid than the brightness alterations in Figure where the depth stratification is absent. A further comparison of these induction effects will be carried out in a subsequent paragraph.The first series of phenomenological observations lead to the following conclusions:(i) The formation of an axis of invariant CP is a source of lightness/darkness induction signals;(ii) Opposite brightness signals give origin to a stratification of the image into layers.An experiment has been designed to assess its consistency and relationship with important factors such as luminance contrast. ExperimentThe test stimuli reproduce Figure. Care was taken to ensure that the reflectance edge separating the two background shades was hidden by the inducers.The method of adjustment was used. The subjects sat at 1 m from the screen, and were asked to observe the configuration on the left (test), compare it with that on the right (comparison), and say whether they perceived differences in luminance between the two sides. They were then asked to adjust the difference in luminance between the vertical bands of the comparison, by clicking on one of two colored arrows at the bottom of the display to reproduce the same difference in luminance they had perceived in the test.
They were instructed to neglect the characteristics of the irregular shapes and concentrate on the luminance of the background.The 18 conditions were presented in random order four times: in two cases the initial comparison stimulus had homogeneous background, or bands of similar shade of gray; in the other two divided background was initially presented. ResultsEach response indicated the luminance difference judged as maximally similar to that of the test stimuli.
In order to obtain a measure of the difference between estimated and real luminance differences, they were subtracted. The simple formula I = (L p-D p) − (L s-D s) here I is magnitude of brightness illusion; L and D indicate lighter and darker luminances; subscript s is subjective value measures how the perceived difference diverges from the real one; I was calculated for each trial and averaged across the four repetitions of the same condition.Results obtained with homogeneous and divided backgrounds underwent separate ANOVA tests on mean values of I. Contrast Polarity Effects on Homogeneous BackgroundFigure shows I-values, obtained with light and dark background, for the three levels of inducer contrast.ANOVA revealed stronger effects with lighter background F (1, 19) = 7.33; p.
(A) The iso-contrast version of the “snake illusion”: the two axes (straight and wavy) have opposite CP as illustrated in the outline patterns on the right. The background between the columns of inducers is traversed by induction signals of the opposite sign. Three elongated ellipsoids drawn in the central portion of the columns serve as targets.
They are identical in gray shade but the central one looks darker. (B) Same as in (A) but with smaller inducers inside the semi-ellipses that generate vertical axes of the opposite sign with respect to (A) as illustrated in the outline on the right. The background is traversed by induction signals of the same sign. In this case the interlaced axes sum their effects. Note the target brightness; the differences are negligible with respect to that observed in (A).
To conclude, one invariant CP axis induces brightness/darkness on the background. If a second set of axes is introduced that propagates induction of the same sign on the background, the effects persists. The targets (in the form of small surfaces) on these portions of the background seem insensitive to the induction signals.Their brightness is found to alter when the two sets of axes propagate on the same portion of the background opposite induction signals.
In this case, when the image splits into a reflectance and an illumination layer, the small target-figures (the diamonds in “snake illusion,” the ellipsoids in Figure ) are perceptually placed at the reflectance layer and darken or brighten in accordance with the induction signals at this level. ConclusionsThe findings reported here confirm the hypothesis that the formation of contours from edges of the same CP generates the brightness/darkness induction effects.
These effects combine in different patterns of interference that have different phenomenological outcomes. For these effects—both binding and induction—to arise it not necessary that four surfaces converge at the same point as seen in the image of an X-junction.
The edges can be non-collinear and their endings can be separated by a gap; in fact if the distance and the deviation of colinearity are within a short range (, ) the effects are clearly visible. This explains why we perceive induction effects even with configurations where the X-junctions are not replicated.Furthermore, on the same area they may propagate induction signals from different combination of axes of the invariant CP, leading to different brightness effects. When the induction signals are of the opposite sign, it is likely that the visual system uses them as cues to decompose the image into layers.Some open questions remain, which should form the basis of a subsequent study.The first question addresses the nature of the induction effects generated by the invariant CP axes. The axes we produced in Figures – are only partially adjacent to the induced surfaces (background) because the “contact edges” alternate with areas of no contact. The conclusion is that the induction signals do not originate along the edges but from a complex unit that integrates shorter edges and becomes a source propagating into the adjacent and non-adjacent surfaces.
Rudd's model (, ) predicting spreading of induction signals beyond edges and strategies of integration offers an account of the findings reported here, but the stimulus conditions of his research differ from those created here so that a complete test cannot be made.The brightness perception is altered at a negligible magnitude or not at all when the luminance of the regions embedded between the invariant CP axes are different (Figure ).This an unexpected finding.It could be one of two things. Il might be that axis 1 does not act as a source of dark/light signals emission. Or it might be that these signals do not combine or sum to the signals (the edge induction signals from the real edges, for example) that allow the observers to give correct judgments when the axes 1 do not complete (Figure ). In other words the induction signals propagating from the illusory contour ( axis 1) cause visible effects on a background of homogeneous luminance but the same effects are not detected when concomitant dark/light signals spread from real high contrasted contours. Previous research on contrast matching does not report findings that can be useful to the understanding of the “anomaly” in Figure findings.The brightness/darkness induction signals from the invariant CP manifest a further property as illustrated in Figure. Here the inducer columns, similar to those of Figure are drawn against a textured background made up of parallel black lines.
IntroductionDevelopmental dyslexia (DD) is often defined as a deficit in reading acquisition despite normal intelligence and access to conventional instruction. According to the dual-route model (see for a review), written words can be processed either by the sub-lexical route or by the lexical route. The sub-lexical route is based on grapheme-to-phoneme correspondences and allows reading of unfamiliar words and pseudo-words. The lexical route is based on lexical unit correspondences and is crucial to read familiar and irregular words only. Both acquired and developmental disorders of reading have been generally discussed within this psycholinguistic framework (e.g., ).
Phonological dyslexics show great difficulty in reading unfamiliar words and pseudo-words compared to known words, and this is thought to arise from damage to the sub-lexical route. In contrast, surface dyslexia is characterized by impaired reading of irregular words, and this is thought to arise from a damaged lexical route (e.g., ), potentially linked to an under-stimulation of the visual word recognition system resulting from low experience with literacy.However, in shallow orthographies such as Italian, spelling-sound irregularity is limited to the supra-segmental level (i.e., to stress assignment). Thus, in Italian dyslexic children the increased weight of sub-lexical processing does not permit precise measurement of the efficiency of the lexical-route (see also ).
It is crucial to note that –- regardless of spelling-sound regularity - for a beginner reader all words are at first pseudo-words because the lexical-orthographic representations still have to be developed. Accordingly, most longitudinal studies have shown that beginner readers primarily use the sub-lexical route (see for a review).Phonological decoding, which is typically measured by examining children’s pseudo-word reading performance, is one of the most critical skills for successful reading acquisition (e.g., ). Interestingly, showed that dyslexics with regular (German-speaking children) and irregular (English-speaking children) spelling-to-sound correspondences present an extremely slow and serial phonological decoding mechanism. Thus, in learning to read it is of utmost importance to acquire an accurate and fluent use of the sub-lexical route (e.g.,; see for a review).Although there are a number of theories attempting to account for DD, two main views received major support. The first approach proposes that DD arises from deficits in systems that are specifically linguistic in nature. In particular, the phonological deficit theory suggests that DD arises from deficits in phonological processing (e.g., ). In contrast, many authors suggest that deficits in underlying non-linguistic sensory mechanisms are the real core abnormality in DD (e.g.,; for visual deficits;; for auditory deficits).
This theory, known as the temporal processing hypothesis is the multi-sensory (i.e., visual and auditory) version of the magnocellular dorsal (M-D) theory of DD, suggesting that children with DD have specific deficits in processing rapidly presented or brief sensory stimuli in either the visual or auditory modalities (see; for reviews). Chiefly, the M-D temporal hypothesis explicitly claims that phonological decoding deficits in dyslexics could arise from impairments in sensory processing of visual and auditory dynamic-stimuli (e.g.,). The well known M-D theory of DD is often referred specifically to the visual modality, and it is a comprehensive, albeit controversial account (e.g.,;; ). This theory stems from the observation that some reading disabled children are impaired in the specific visual M-D pathway (see;; for reviews). The M-D pathway originates in the ganglion cells of the retina, passes through the M-layer of the lateral geniculate nucleus (LGN), and finally reaches the occipital and parietal cortices. The M-D stream is considered blind to colors, and responds optimally to contrast differences, low spatial frequencies, and to high temporal frequencies and motion.
The M-D stream seems to be impaired in individuals with DD, whereas the other major parallel pathway of the visual system, the parvocellular-ventral (P-V) stream, is intact (see;; for reviews). The P-V pathway is characterized by both lower temporal resolution and superior sensitivity to high spatial frequencies, and it is also sensitive to color changes. Several studies showed the specificity of the M-D pathway deficit in individuals with DD in comparison to P-V processing in the normal range, suggesting the crucial role of the M-D pathway as the dominant visual stream for text reading (e.g.,; ).
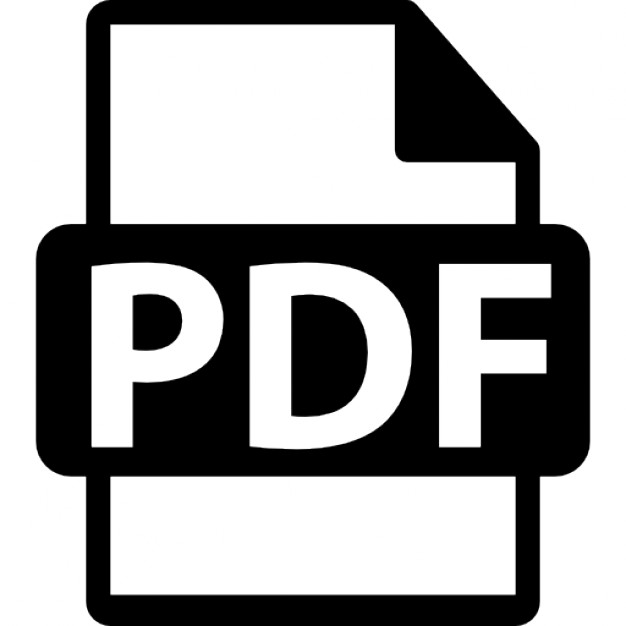
All participants underwent a complete ophthalmological evaluation, consisting of “Early Treatment Diabetic Retinopathy Study” (ETDRS) chart (standardized eye charts and visual acuity test), orthoptic examination, anterior segment slit lamp examination, cycloplegic refraction, and indirect ophthalmoscopy. Apparatus and stimuliFrequency doubling perimetry. The FD perimetry relies on the frequency doubling illusion described in the Introduction. The settings resembled the ones adopted, in a previous study,.
A low spatial frequency grating displayed in counter-phase flicker mode at a high temporal frequency is perceived as if it had twice its actual spatial frequency. The Humphrey Matrix perimeter was the presentation tool used with the program threshold set to 30-2. The threshold is expressed as contrast attenuation in decibels (dB) and it is calculated by a staircase algorithm built into the Humphrey Matrix perimeter tool.
Thresholds ranged from 0 to 38 db. The stimulus was presented at 69 locations in the 30 central degrees of the visual field.
The background luminance was 100 cd/m 2. The pattern consisted of a sinusoidal grating presented at different contrast levels, arranged in 5° × 5° square stimuli, and a circular macular stimulus of 2.5° radius.
The spatial frequency of the bar target was 0.50 cy/deg, the counter-phase flickered at 18 Hz and was presented for 300 ms. The FD provides selective stimulation of the M-retinal ganglion cells and M LGN neurons. Due to the design of the target, no P-cell activity should be stimulated.
Visual fields with 20% or less false positive or false negative responses, and 30% or less fixation errors were considered acceptable.High-pass resolution (ophthimus) perimetry. The Ophthimus system HPR perimetry uses ring-shaped stimuli, consisting of dark borders and a lighter core. Fourteen different sized targets are available (ranged from 1.26 to 17.64 dB).
The target contrast was held constant while the size varied in steps of 1.26 dB. The background luminance was 20 cd/m 2.
The luminance of the ring borders was 15 cd/m 2 and the luminance of the ring core was 25 cd/m 2. The target was “high-pass spatial frequency filtered”. The participants either detected and resolved it, or it was invisible to them.
The perimeter assesses resolution thresholds as the smallest stimulus size seen in the 50 locations over the central 30° of the visual field. The blindspot is not mapped. The high-pass spatial frequency filter allows for selective analysis of the P-cells of the retina and probably of the LGN. Due to the software characteristics, fixation errors were not tested for the HPR perimetry. ProceduresAll participants performed the FD and the HPR perimetry in random order, beginning with one of the two eyes. On another day, they performed the two visual field tests again, beginning with the other eye, in order to avoid fatigue and any learning effects. The children were verbally instructed on how to perform the two tests and were given the opportunity to practice.
Two pauses were given throughout each test, and a 5-min pause was permitted between testing of the first and second eye.Each child was seated comfortably with their face against the eyepiece. For the FD testing the child was given a description of the display, and instructed to press the response button each time she/he saw a pattern against the homogeneous background. For the HPR task each child had to report whenever they saw a circle in any tested position of the visual field.
Results FD resultsAll groups and subgroups were normally distributed as showed by a non-significant Shapiro-Wilk test of normality (all ps.05). The mean FD thresholds (averaged for all positions and the two eyes) for the children with DD ( n = 17) differed significantly t-test: t (39) = 2.697, p 0.05, η p 2 = 0.068. In summary, only the PPDs were significantly worse than the controls in their mean FD threshold. Based on these results indicating a specific relationship between M-D pathway and the spelling to sound translation process we concentrate our Experiment 2 only on the PPDs. HPR resultsAll groups and subgroups were normally distributed as showed by a non-significant Shapiro-Wilk test of normality (all ps 0.05). The mean HPR thresholds (averaged for all positions and the two eyes) for the children with DD ( n = 17) and the normal reader age- and IQ-matched controls ( n = 24) did not differ significantly t-test: t (39) = 0.432, p 0.05 showing that the dyslexic and normal reader groups were similar in their P-V pathway performance (see Figure and the plot of the individual data in Figure ).
The relationship between M-D functioning and reading text abilityPartial correlation between M-D pathway functioning (indexed by FD threshold) and the text reading efficiency (the mean between speed and errors z-scores) in the entire sample ( n = 40; a child with DD score is missing), controlling for chronological age, IQ (block design and similarities), and the P-V functioning, was significant ( r = 0.43, p. Apparatus and stimuliFrequency doubling perimetry. The procedure was exactly the same for the Experiment 1. The HPR perimetry task was not performed because the results were not be discriminative in the Experiment 1. ResultsThe mean FD thresholds (averaged for all positions and the two eyes) for PPDs ( n = 8) and the typical readers IQ- and RL matched controls ( n = 10) differed significantly t-test: t (16) = 2.962, p 0.05).
It is not surprising that age did not affect that task because the M-D pathway should be completely operative much before the age of 7 years (e.g., ). (A) The scatter-plot of the individual data showing the difference between the children without DD (i.e., CA and RL controls) and the subtypes of children with DD (NPD and PPD) in the FD task measuring the M-D pathway functionality (Experiment 1 and 2).
The findings did not change running the ANCOVA without the outliers. Error bars represent one standard deviation of the mean. (B) The plot of the individual data showing the difference between the children without (i.e., CA controls) and with DD in the HPR task (Experiment 1). Error bars represent one standard deviation of the mean. Although the study was designed as two independent experiments the PPDs of the Experiment 1 and 2 were very similar in age and they did not differ in other relevant variables. Additionally, we found it could be of interest to merge the two groups of PPDs and contrast them with the CA and the RL controls. We applied an univariate ANOVA with the FD threshold as the independent variable and groups (PPD, n = 25, CA, n = 24 and RL, n = 10) as the between subjects factor.
The Group main effect was significant F (2,56) = 7.33; p.